Where I come from, we call it a “Discovery Flight.” It’s a short flight that gives a non-pilot an introduction to the flight school and flying in general. “You’ll take the controls!” is a time-honored way for flight schools to market the flights, which I really enjoy doing. Some of the customers have never been in an airplane at all, and most have never been in one as small as a Cessna 172. We’re supposed to fly for 0.5 on the Hobbs, but I usually stretch it to 0.6. It’s a lot of fun to see the joy that people get from their first ride in a personal airplane and, perhaps, their first-ever flight. Now the shoe is on the other foot: I am as new to Aviation Safety as these customers are to the art of flying airplanes. What’s the first thing that I should tell anyone, you or them, about flying? There are so many tempting topics.
Most of my recent flying has been in a Beech King Air B200 equipped with Garmin G1000 avionics. I love to talk about this stuff with pilots, but it won’t help non-pilots who are about to handle the controls for the first time. They don’t need the in-depth training required for the Robinson R-22 in SFAR 73, but if they don’t have some idea about what the controls do, they won’t have as much fun. And they’re less likely to come back for a second flight, much less sign up for lessons.
The most basic maneuver in flying is the level turn. It’s what separated the Wright Brothers from maple seeds: they controlled the craft. So that’s the only maneuver I have newcomers do on their Discovery Flight. After finishing their training, pilots and even instructors sometimes forget what an intricate maneuver this is. We also forget that a botched turn at low speed and low altitude can be deadly. Look at the 2018 Nall Report from the AOPA Air Safety Institute. The biggest accident category for non-commercial fixed-wing airplanes was “landing,” and there were 177 “loss of control” accidents and 52 “stall” accidents. It’s a maneuver that has to be done right. (If you don’t agree, please see the article beginning on page 4, “Moose Stalls,” then return. — Ed.)
HOW TO TURN AN AIRPLANE
On the Discovery Flight, we don’t just get in the airplane and go. We discuss the fun stuff like which route to take, and we discuss the basic aerodynamics of turning flight. I don’t call it that, but that’s what it is. I only have five minutes or so to explain flight, a little more if the customer is an engineer or something similar, a little less if the customer is in middle school. It has to be concise, complete and entertaining.
A lot of non-pilots say that rudder makes an airplane turn. People with more experience do better and say that ailerons make an airplane turn. Those are the two principal flight controls used in a turn, but why are they there, and how do they make the airplane turn?
What makes an airplane turn is the same thing that makes it fly: lift. Basic physics says that lift is a force, and if you want anything to change its path you must apply a force. When the airplane is in a bank, some of the lift is directed to the side (textbooks talk about the “horizontal component of lift”). To get an airplane to turn, you need to put it into a bank so that some of the lift is directed to the side.
In my opinion, this is the most fundamental and important fact about flying.
The two diagrams at right are typically used to explain what’s going on in level turns and, among other things, why load factor and stalling speed both increase. One of the keys is understanding that these values exist in all turns—level, descending or climbing—but their magnitude depends on airspeed and bank angle, among other factors.
Another key is learning to manage aileron, rudder and pitch to ensure the turn is, indeed, a level one. At steeper angles of attack and greater speeds, more rudder and more nose-up pitch input is required. The converse is true when slower and less steeply banked.
ADVERSE YAW
One question often arises: “How do you get an airplane into a bank?” As we both know, the answer is to use the ailerons. Ailerons make the airplane roll, and on the ground I demonstrate with an imaginary yoke and roll my body to the side. “For a right turn, start with right aileron until the airplane is in the proper bank, then neutral, then left aileron to level the wings.
“Got all that? Okay, let’s go try it!” I say. I start to get up, but if it appears the customer is ready for more, I say, “Wait, it’s not that simple.”
I stick my arms out to the side and mimic the action of the ailerons with my hands. The wing going down needs no help—gravity is its friend—but the wing going up has to fight gravity. I remind them that when you stick your hand out the window of a moving car and tilt it up, the wind forces it up while it also forces it back. I take a little model of a 172 like the one we’ll fly and show how adverse yaw pulls the nose of the airplane to the left, the side of the down aileron for a right turn.
“That’s what the rudder is for,” I add. I grab the model airplane by the vertical stabilizer and let it hang with its nose pointing at the floor. “The rudder moves the nose left or right. You do that with the pedals.” Then I stick my feet on a chair and mimic kicking while pulling on the stick or yoke. The airplane needs rudder while it’s rolling. As soon as the ailerons are neutralized, the adverse yaw disappears. “Okay, let’s go try it!” I repeat.
LIFT AGAIN
Once again, I start to get up, but stop. “Wait, it’s not that simple.” The problem is that with so much lift directed to the side there isn’t enough to hold the airplane up. You can go into more detail but that’s a good first explanation. In the turn, the airplane needs more lift, and you can get a little more lift with the elevators. I remind them that in Amelia Earhart’s day, these were called the “flippers,” and I kind of wish we would go back to that terminology.
Once the airplane is in a bank, it needs more elevator because it needs more lift. You know that this can be dangerous, because pulling too hard increases the angle of attack beyond the critical one and the wing will stall, but that’s too complicated for a first lesson. Instead of telling the customer how hard not to pull, I have them hold a pen or pencil and say, “You have to pull about that hard.” It is, after all, a matter of pressure on the controls at this point.
So, there it is: All of the conventional flight controls have a purpose, and I have explained that purpose to them.
Aileron, rudder and elevator aren’t the only way to make an airplane turn. One obvious example is the V-tail Bonanza. Another is the Cirrus Aircraft SF50 Vision Jet, pictured at top. The Bonanza’s tail has two ruddervators that affect both pitch and yaw. One of the advantages is that instead of a vertical stabilizer and left and right horizontal stabilizers, the Bonanza only has two surfaces on the tail. That means less drag.
The Mitsubishi MU-2 has a conventional rudder and elevator, but uses spoilers for roll control. That means no adverse yaw. So, why did it need a rudder at all? The first reason is that the rudder was still needed to control P-factor, or left-turning tendency, from its two powerful turboprop engines. The second was for aircraft control after an engine failure. The rudder compensates for the asymmetric thrust.
In a twin-engine airplane, an engine failure generally requires almost full rudder. If less rudder input was needed, the designers would have made the tail smaller, saving weight and drag and resulting in a faster airplane with more useful load.
Some aircraft, like the Horten HO-229, the Northrop YB-49 and the Northrop Grumman B-2, pictured at bottom, have no tail at all. The Horten and Northrop flying wings used elevons for yaw control. The B-2 uses complex computer algorithms that include differential thrust. Sticking an elevator or other control surface into the slipstream increases the aircraft’s radar cross-section, negating its stealthy features.
P-FACTOR
Depending on the customer, I might also get a chance to talk about P-factor, or left-turning tendency. It’s a judgment call, depending on the customer’s background and inclination.
P-factor can be a challenge to explain because it is difficult for some to visualize that the downward blade and upward blade have different angles of attack. The difference comes from the forward movement of the airplane. Whether you can visualize it or not, you know it happens. The engine at high power generates a lot of left-turning tendency at any speed, but the ability of the tail surfaces to compensate is much lower at slow airspeeds. That’s why you need right rudder in a climb. (I once got to fly a de Havilland Tiger Moth, whose engine rotates the other way. That generates a right-turning tendency, so in the climb it needs left rudder.)
Newcomers ask how much rudder to use, but anticipating that depends on weight and CG. With an aft CG, you need more rudder because the rudder’s arm is shorter. A practical way to learn this is to point the climbing airplane at a cloud and experiment using the pedals, keeping the cloud at the same spot on the windshield.
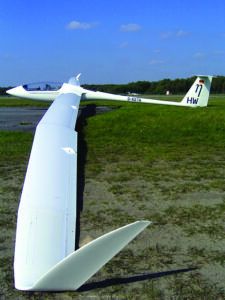
Gliders (or sailplanes if you prefer) don’t really make level turns because they are always descending. I have talked with experienced glider pilots who struggled with this when transitioning to powered flight, who then realize, “I’ve never made a level turn in my life!”
When a glider is thermalling, it is making a descending turn, but it is doing so in air that is rising faster than the glider is sinking. This is the vertical version of the relationship between true airspeed and groundspeed. Now, instead of a goldfish swimming across the bowl while you carry the bowl across the room, the ball is rolling down an inclined plane in a rapidly climbing elevator.
The glider still needs some back pressure in the turn in order to stay at equilibrium. Three of the four forces still apply—lift, gravity and drag, but power is missing, replacing kinetic energy with potential energy. They also tend to need a lot more rudder input than most powered fixed-wing aircraft. That’s due to adverse yaw being more of a factor because the wings are so long, airspeeds are low—airflow over the tail typically is much less than an airplane—and the vertical stabilizer is usually as small as possible.
SECONDARY CONTROLS
Secondary controls include flaps, slats and spoilers. Some aircraft, like the 1946 Taylorcraft BC-12D I owned, have none, and when I had it, I got to be really good at slipping. I’ve never flown with slats; they’re not common on GA airplanes.
Most gliders have speed brakes that act a lot like spoilers. They almost feel like a throttle. The technique with speed brakes is to fly from the abeam point on downwind with them about half open. If the glider is getting low, you push on the spoiler control to close them and accelerate, just like you would with a throttle. This doesn’t increase thrust, it reduces drag, but when looking at the four forces in total, this has the same effect.
A big advantage of spoilers and speed brakes is that they have no noticeable effect on yaw. Slipping is an alternative.
Flaps change the airflow and almost always lead to a pitch change. In Cessna singles and many other types, lowering flaps raises the nose. I have students slow to a speed in the white arc and select full flaps, which makes the nose rise dramatically.
In Piper singles with manual flaps, the pitch change may or may not be in the same direction as the Cessnas, depending on whether they have the “Hershey Bar” wing or the more modern tapered wing. Either way, the pitch change can happen a lot faster. Going from full flaps to none in zero time can float everything in the cabin.
FINAL THOUGHTS
I’ve been looking into adding on a seaplane rating and have visited to dozens of seaplane training sites. One of the frequent comments is that it is important for pilots to understand what happens when you move the controls. Land pilots can see it’s important while taxiing a high-wing airplane downwind on a windy day—the aileron on the upwind side must be down to keep that wing from being lifted, possibly leading to an upside-down airplane. And that’s one more reason you should check that the controls are correct before starting to move.
Jim Wolper is an airline transport pilot and mathematics professor. He’s also a CFI with single-engine, multi-engine, instrument and glider ratings.
Explaining what we do to control the airplane gets very complicated very quickly. An analogy to the factors that go into a turn, ailerons and rudder, consider a bicycle. How many of us grew up riding bikes and turning without thinking of it? If we think of the ailerons as leaning the airplane into the turn, then the two are very similar. You can’t turn a bike just by the steering wheel, and you can’t turn a bike just by leaning it over, so both steering (think rudder) and leaning (think ailerons) are required. Maybe this would help explain to a new-comer why both are necessary. Just a thought.
nice explanation of both your technique AND the small bites you lead your potential customers through some very complex material. kudos